The Principles of Laser Generation
Lasers, due to their unique physical properties, are often referred to as "the fastest knife," "the most accurate ruler," and "the brightest light."
Why Know the Principles of Lasers?
Understanding the differences among common semiconductor lasers, fibers, discs, and YAG lasers can provide more insight when choosing models and also offer more topics for discussion. This article is primarily educational, aiming to briefly introduce the principles of laser generation, the main structure of lasers, and several common types of lasers.
Principle of Laser Genertion
The English word "laser" is derived from the acronym for "light amplification by stimulated emission of radiation," which means the amplification of light through stimulated emission of radiation. Initially, the Chinese translation of "laser" was phonetically transcribed as "镭射" (which is still used in regions such as Hong Kong and Taiwan of China), but later, under the advocacy of the leading figure in science and technology, Qian Xuesen, it was uniformly referred to as "激光" in mainland China.
Lasers are produced through the interaction between light and matter, that is, the so-called light amplification by stimulated emission of radiation. Understanding this process requires knowledge of concepts proposed by Einstein, such as spontaneous emission, stimulated absorption, and stimulated emission, along with some necessary theoretical foundations.
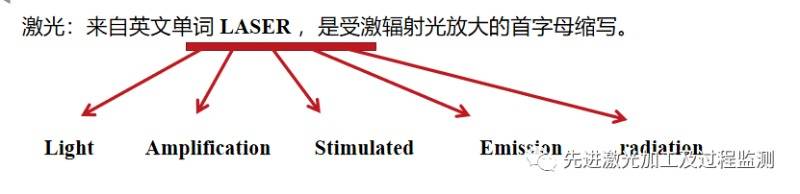
image credit: Willdong
Theoretical Foundation I: The Bohr Model
The Bohr model primarily provides an understanding of the internal structure of atoms, facilitating comprehension of how lasers are generated. Atoms consist of a nucleus and electrons orbiting around it. The orbits of the electrons are not arbitrary; there are only specific orbits available to them. The innermost orbit is referred to as the ground state; when an electron is in the ground state, its energy is at its lowest. If an electron jumps to an outer orbit, it is said to be in the first excited state, which has a higher energy level than the ground state; moving to yet another outer orbit puts the electron in the second excited state.
The generation of lasers is possible because electrons move between these different orbits within the model. If an electron absorbs energy, it can move from the ground state to an excited state; conversely, when an electron falls back from an excited state to the ground state, it releases energy, which is often emitted in the form of a laser.
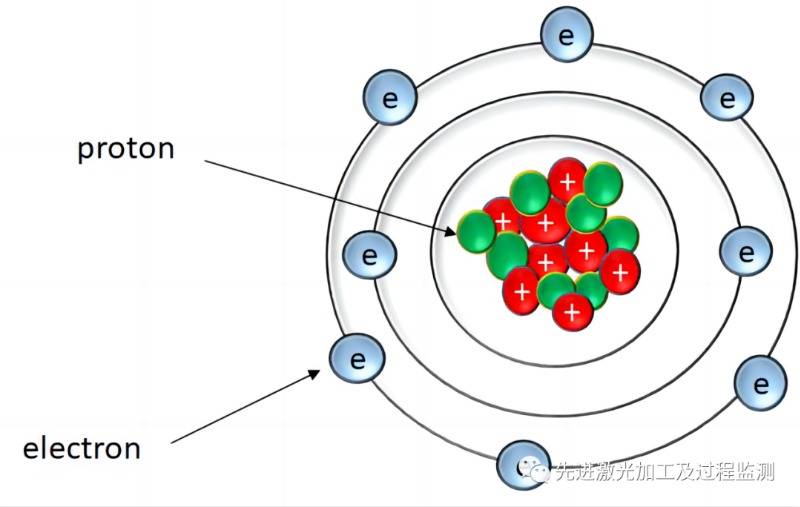
image credit: Willdong
Theoretical Foundation II: Einstein's Theory of Stimulated Emission
Spontaneous Emission
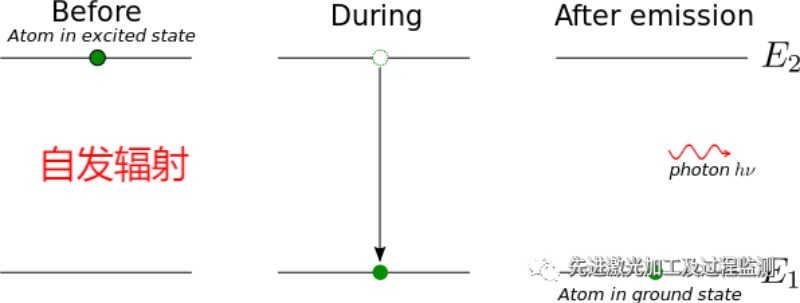
image credit: Willdong
As depicted: an electron at a higher energy level, E2, spontaneously transitions to a lower energy level, E1, and emits a photon with energy hv, where hv=E2-E1. This spontaneous transition process, which is independent of external influences, is known as spontaneous emission, and the light waves emitted during this process are considered spontaneous radiation.
Characteristics of Spontaneous Emission: Each photon is independent, with different directions and phases, and the timing of emission is random, leading to incoherent light that is chaotic and not what is desired for lasers. Therefore, the laser generation process aims to reduce this type of stray light. This is one of the reasons for the presence of stray wavelengths in laser devices. With proper control, the proportion of spontaneous emission in laser devices can be negligible, leading to purer lasers. For example, if a laser is specified as 1060, it means all emitted wavelengths are exactly 1060nm, resulting in a laser with more stable absorption rates and power output.
Stimulated Absorption
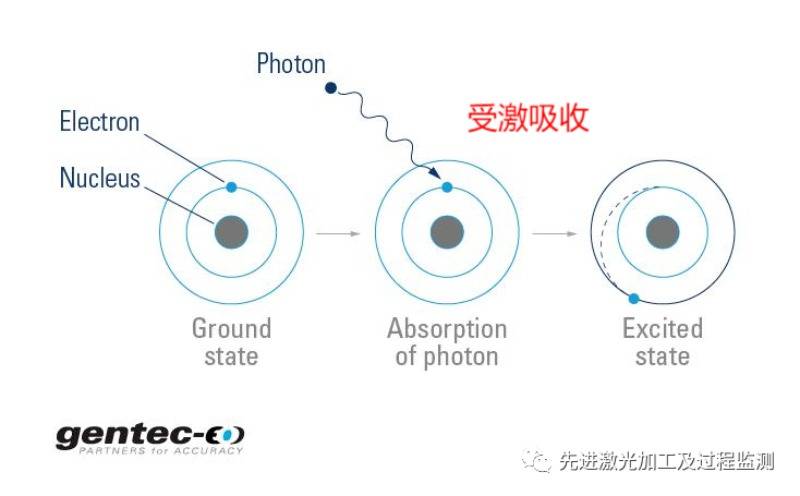
image credit: Willdong
When an electron at a lower energy level (lower orbit) absorbs a photon and transitions to a higher energy level (higher orbit), this process is called stimulated absorption. Stimulated absorption is crucial; it is one of the key processes in pumping, where the pump source provides photon energy to cause particles in the gain medium to transition to higher energy levels, awaiting stimulated emission to produce laser light.
Stimulated Emission
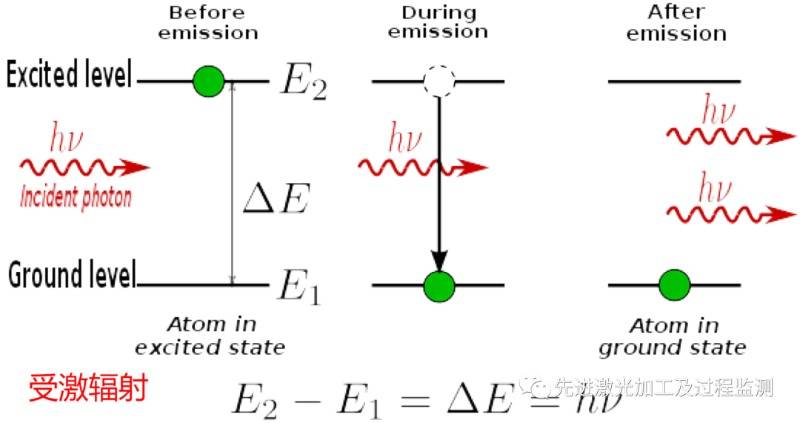
image credit: Willdong
When exposed to external energy (hv=E2-E1) light, an electron at a higher energy level is stimulated by the incoming photon to transition to a lower energy level (from a higher orbit to a lower orbit), and in the process, it emits a photon identical to the incoming photon. This process does not absorb the original stimulating light, resulting in two identical photons. It can be understood as the electron releasing the previously absorbed photon, a process known as stimulated emission, which is the reverse process of stimulated absorption.
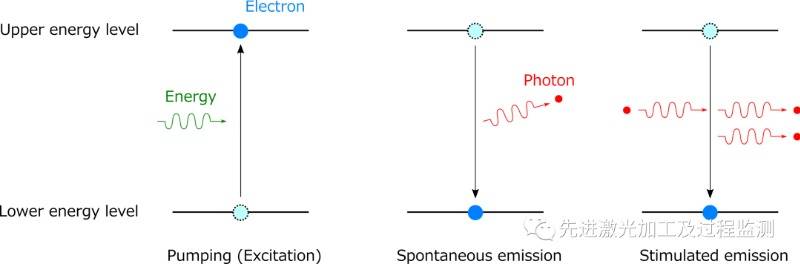
image credit: Willdong
With a clear understanding of the theory, building a laser becomes straightforward. As depicted, under normal conditions, the vast majority of electrons are in the ground state. Laser generation relies on stimulated emission, so the structure of a laser is designed to first induce stimulated absorption, elevating electrons to higher energy levels. Then, with stimulation, a large number of high-energy electrons undergo stimulated emission, releasing photons. This process generates laser light. Next, we will introduce the structure of a laser.
Structure of a Laser
The structure of a laser corresponds directly with the conditions required for laser generation as discussed previously:
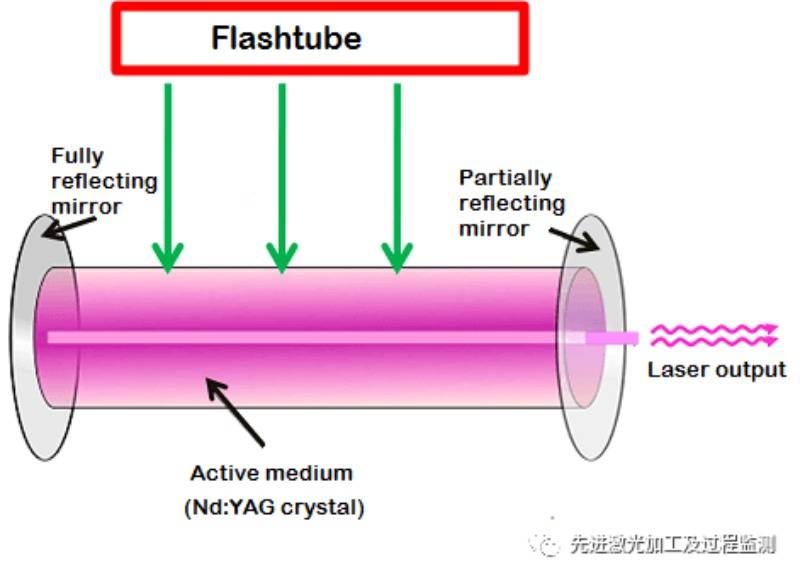
image credit: Willdong
A gain medium serves as the working medium for the laser, with activated particles possessing the energy level structure suitable for producing stimulated emission (mainly capable of pumping electrons to high-energy orbits and maintaining them there for a certain duration before releasing photons through stimulated emission all at once).
An external excitation source (pump source) can pump electrons from lower to higher energy levels, creating a population inversion between the laser's upper and lower energy levels (where there are more particles at the higher energy level than at the lower one), such as the xenon lamp in a YAG laser.
A resonant cavity to achieve laser oscillation, which extends the working length of the laser material, filters the modes of light waves, controls the direction of beam propagation, and selectively amplifies the frequency of stimulated emission light to enhance monochromaticity (ensuring the laser reaches a certain energy before output).
Corresponding structures are illustrated as in the diagram of a basic YAG laser configuration; others may be more complex, but the core principle revolves around the laser generation process as shown in the diagram.
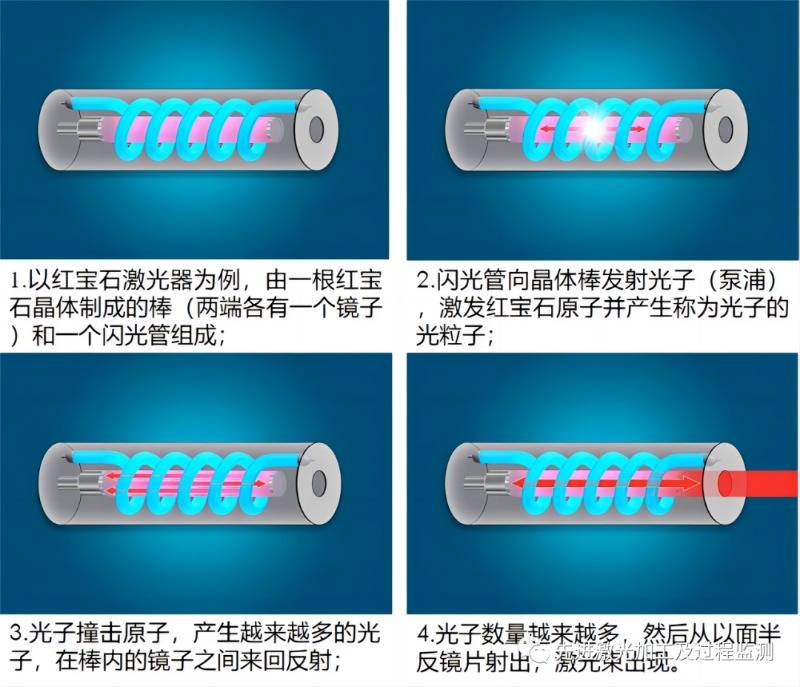
image credit: Willdong
Classification of Lasers
1. Based on the Gain Medium
Carbon Dioxide Lasers
The gain medium for carbon dioxide lasers is a mixture of helium and CO2, producing a laser wavelength of 10.6um. These were among the earliest lasers to be commercialized, initially dominating laser welding applications. Currently, they are primarily used for welding and cutting non-metallic materials (such as fabrics, plastics, wood, etc.) and in lithography machines. Carbon dioxide lasers cannot be transmitted through optical fibers and rely on free-space optical paths, finding extensive use in cutting equipment.
YAG (Yttrium Aluminum Garnet) Lasers
Utilize a YAG crystal doped with neodymium (Nd) or ytterbium (Yb) metal ions as the laser gain medium, emitting a wavelength of 1.06um. YAG lasers can produce high pulses but have a lower average power, with peak power reaching up to 15 times the average power. They are primarily pulse lasers and cannot maintain continuous output. However, they can be transmitted through optical fibers and have increased absorption rates in metal materials, initially finding applications in reflective material processing and the 3C (Computer, Communication, and Consumer Electronics) sector.
Fiber Lasers
The current mainstream in the market, using ytterbium-doped fiber as the gain medium, with a wavelength of 1060nm. Depending on the shape of the medium, they can be further divided into fiber and disc lasers.
Semiconductor Lasers
The gain medium is a semiconductor PN junction, with the wavelength of semiconductor lasers primarily at 976nm. Currently, near-infrared semiconductor lasers are mainly used in cladding applications, with beam spots exceeding 600um.
2. Classification by Energy Interaction Mode
Pulsed Lasers
Operating in nanoseconds, picoseconds, and femtoseconds, these high-frequency pulsed lasers (ns indicating pulse width) can achieve high peak energy and high frequency (MHz) processing. They are used for thin-plate copper and aluminum processing of dissimilar materials and cleaning, benefiting from their high peak energy for rapid melting of the base material, short interaction times, and minimal heat-affected zones, providing advantages in processing ultra-thin materials (below 0.5mm).
Quasi-Continuous Wave Lasers (QCW)
Quasi-continuous wave lasers, with high repetition rates and low duty cycles (below 50%), have pulse widths ranging from 50 µs to 50 ms. Quasi-continuous fiber lasers bridge the gap between kilowatt-level continuous fiber lasers and modulated pulse lasers; their peak power can reach up to 10 times the average power of continuous mode operation. QCW lasers typically have two modes: low-power continuous welding and high-peak power pulse laser welding at 10 times the average power, allowing for welding thicker materials and more heat while controlling the heat within a small range.
Continuous Wave Lasers (CW)
These are the most commonly used lasers, with most of the lasers seen on the market being continuous wave. They provide continuous laser output for welding and processing. Fiber lasers, depending on their core diameter and beam quality, are divided into single-mode and multimode lasers, adaptable for different application scenarios.